Introduction
The Multi-Level Perspective (MLP), which has become the dominant conceptual approach in sustainability transitions research (Hansmeier et al., 2021), focuses on transitions in socio-technical systems (such as energy, transport, housing, and agri-food systems), which are the prime drivers of persistent sustainability problems such as climate change, biodiversity loss, and resource depletion (EEA, 2019). To understand the dynamics of sustainability transitions, the MLP distinguishes three analytical levels. At the first level, radical ‘green’ innovations that form the seeds of sustainability transitions emerge in protected niches. Table 1 provides some examples with varying degrees of maturity and radicality.
Mobility | Agri-food | Energy (electricity, heat) | |
Radical technical innovation | Battery-electric vehicles, (plug-in) hybrid electric vehicles, biofuel cars; hydrogen cars | Permaculture, agroecology, artificial meat, plant-based milk, manure digestion | Renewable electricity (wind, solar, biomass, hydro), heat pumps, passive house, biomass stoves, smart meters |
Grassroots and social innovation | Car sharing, bike clubs, modal shift to bicycles and buses, tele-working, tele-conferencing | Alternative food networks, organic food, less-meat initiatives, urban farming | Decentralized energy production (‘prosumers’), community energy, energy cafés |
Business model innovation | Mobility services, car sharing, bike sharing | Alternative food networks, organic food | Energy service companies, back-up capacity for electricity provision, vehicle-to-grid electricity provision |
Infra-structural innovation | Intermodal transport systems, compact cities, revamped urban transport systems (tram, light-rail, metro) | Efficient irrigation systems, agroforestry, rewilding, multi-functional land-use | District heating system, smart grids, bio-methane in reconfigured gas grid |
Table 1: Examples of radical niche-innovations in mobility, agri-food and energy domains (Geels, 2019: 190)
Green niche-innovations face uphill struggles against existing unsustainable systems and the associated rules and institutions (which are called ‘regimes’), which form the second level. These systems and regimes are difficult to change because they are entrenched and stabilised by various lock-in mechanisms (Geels, 2004; Klitkou et al., 2015). These include techno-economic lock-in mechanisms such as sunk investments (in plants and infrastructure), low cost (because of scale economies) and high performance (because of decades of learning-by-doing improvements); social and cognitive lock-in mechanisms such as routines and mind-sets that blind actors to developments outside their focus (Nelson, 2008), social capital resulting from long-standing relations between actors, and user practices and life styles that have become organised around particular technologies; and political lock-in mechanisms such as existing regulations that favour incumbents (Walker, 2000) and lobbying efforts by vested interests to maintain the status quo and hamper radical innovation (Geels, 2014).
The third level, called the socio-technical landscape, captures broad exogenous developments that shape niche and regime developments, either through rapid shocks (such as wars, recessions, pandemics) or gradual changes (such as macro-economic, macro-cultural, or geopolitical trends) that exert pressures or create favourable contexts.
The MLP explains transitions as resulting from developments within and between these three levels, which are always enacted by multiple social groups, as later sections elaborate. The MLP has become an attractive and widely-used middle range theory for many sustainability transitions scholars because of its approach to several foundational social science sustainability debates.
Its meso-level systems focus enables the MLP to overcome the unfortunate dichotomy in other sustainability approaches that either have a macro-focus (aimed at changing capitalism or consumerism) or a micro-focus (aimed at changing individual behaviour, attitudes, and motivations) (Geels et al., 2015).
Its conceptualisation of systems as socio-technical configurations, involving alignments between technologies, consumer practices, cultural meanings, governance arrangements, business models, markets, and infrastructures (Geels, 2004), further enables the MLP to overcome the long-standing dichotomy between behaviour change or technical change as sustainability transformation strategies.
The MLP’s focus on actors and social groups (e.g., firms, consumers, social movements, policymakers, researchers, investors), who act and interact in the context of (gradually changing) rules and institutions, enables it to accommodate recursive interactions between both agency and structure (Geels, 2004).
And the MLP’s attention for both lock-in mechanisms of existing systems and the emergence of radical innovations in niches, enables it to accommodate both stability and change. Lastly, the MLP’s attention for unfolding processes at different levels enables it to accommodate different timescales (Geels, 2022), which resonates with Braudel’s (1970) approach to explaining long-term historical processes as involving slow-changing developments (‘longue durée’), conjunctural developments and cycles, and short-term (smaller and bigger) events (Figure 1).
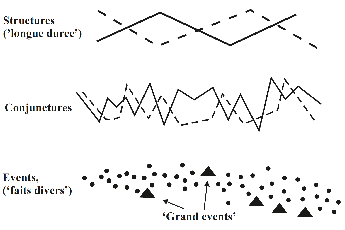
Section 2 of this chapter further describes the MLP’s basic concepts and historical backgrounds. Section 3 provides a brief empirical illustration. And section 4 addresses ongoing debates and current research topics.
2. Historical background and overview of basic concepts
The Multi-Level Perspective emerged from the Twente school’s quasi-evolutionary model of technological development (Rip, 1992; Schot, 1992), which combined concepts from evolutionary economics (e.g., search routines, innovation, selection environment, technological regimes) and sociology of innovation (e.g., social interactions and networks, cognitive interpretations, co-construction of technology and society). Drawing on these concepts, Rip and Kemp (1996) developed a basic MLP-version to understand the biography of radical innovations as emerging in small niches, followed by selection and uptake into existing technological regimes, and finally becoming part of a slowly evolving socio-technical landscape (Figure 2).
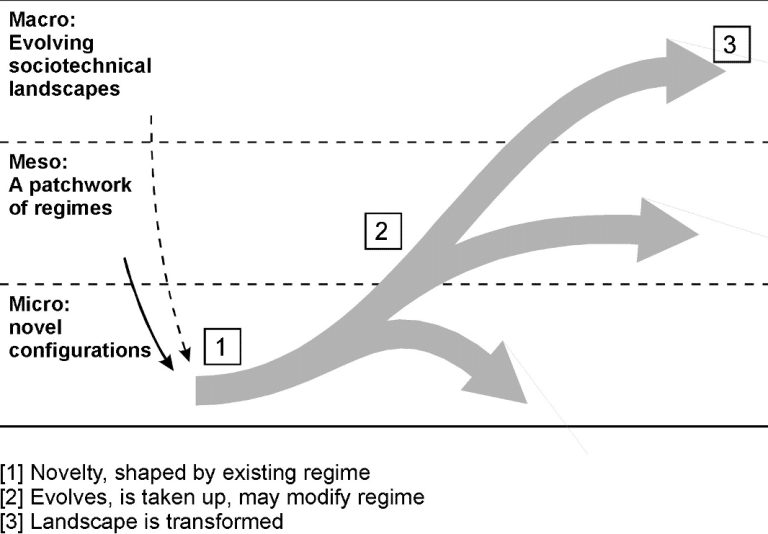
Shifting the focus from bottom-up innovation journeys to socio-technical system transitions, Geels (2002) elaborated this into a more full-fledged Multi-Level Perspective, which broadened the focus from technological to socio-technical regimes and placed greater emphasis on the alignment of emerging niche-innovations with ongoing developments at regime and landscape levels (Figure 3). This full-fledged MLP conceptualises socio-technical transitions as progressing through four phases.

In the first phase, radical innovations emerge in small niches at the periphery of existing systems, through pioneering activities of entrepreneurs, start-ups, activists or other relative outsiders (Schot and Geels, 2008). Niches form ‘protected spaces’ that provide shelter from mainstream market selection and nurture the learning and development of radical innovations (Smith and Raven, 2006). Meanwhile, developments in the existing system and regime continue through incremental adjustments along predictable trajectories (represented as straight lines in Figure 3).
In the second phase, radical innovations establish a foothold in one or more market niches, which provides a more reliable flow of resources. Learning processes gradually stabilise the innovation into a dominant design, which becomes institutionalized in product specifications, design guidelines and best practice formulations (Geels and Raven, 2006). Niche-innovations face up-hill struggles against deeply entrenched systems, which continue to develop along incremental trajectories because of stabilising lock-in mechanisms. The niche-innovations are still more expensive than existing technologies and there may be deep uncertainties about users and their specific preferences (Oudshoorn and Pinch, 2003).
In the third phase, the innovation diffuses into mainstream markets, where it competes head-on with the existing system. On the one hand, diffusion depends on niche-internal drivers such as price/performance improvements, scale economies, development of complementary technologies, and support from powerful actors (Geels and Johnson, 2018). On the other hand, diffusion depends on external landscape developments that pressure the regime, leading to tensions and an ‘opening up’ of the regime (represented by diverging arrows in Figure 3). The diffusion phase is often characterized by heated multi-dimensional struggles, including business struggles between new entrants and incumbents, which may lead to the downfall or reorientation of existing firms (Penna and Geels, 2015), political struggles over adjustments in policy goals and policy instruments such as subsidies, taxes, and regulations (Meadowcroft, 2009), and discursive struggles about the framing of problems and solutions and the rationales for action or inaction (Roberts and Geels, 2018).
In the fourth phase, the new socio-technical system replaces the old one, and becomes anchored and institutionalized in regulatory programs and new agencies, habits of use, views of normality, professional standards, and technical capabilities. System transitions are not about single technologies (e.g., renewable energy), but also involve complementary innovations (e.g., smart meters, energy storage), infrastructure adjustment (e.g., smart grids, bi-directional flows), new business models (e.g., capacity markets), and user practices (e.g., demand response, self-generation) (Geels and Turnheim, 2022).
3. Empirical application: The German electricity transition (1986-2022)
Early MLP conceptualisations have been criticised for being unclear about the operationalisation of core concepts such as ‘regime’ for empirical research (Genus and Coles, 2008; Holtz et al., 2008). Scholars therefore subsequently more clearly distinguished the socio-technical system (as consisting of interacting tangible elements) from the socio-technical regime (consisting of rules and institutions), and reconceptualised the latter using ideas from neo-institutional theory (such as regulative, normative, and cultural-cognitive institutions) rather than from Giddens’s structuration theory (Fuenfschilling and Truffer, 2014; Geels, 2020b). To make the MLP’s basic ideas more concrete, this section applies the MLP to the German electricity transition (Geels et al., 2016). Electricity from renewable energy technologies (RETs), which includes niche-innovations such as solar-PV, biomass, and wind turbines, increased from 3.6% in 1990 to 44.6% in 2022, while regime technologies such as nuclear energy, brown coal (lignite) and hard coal declined substantially (Figure 4). Natural gas increased until 2010 and has fluctuated since then.
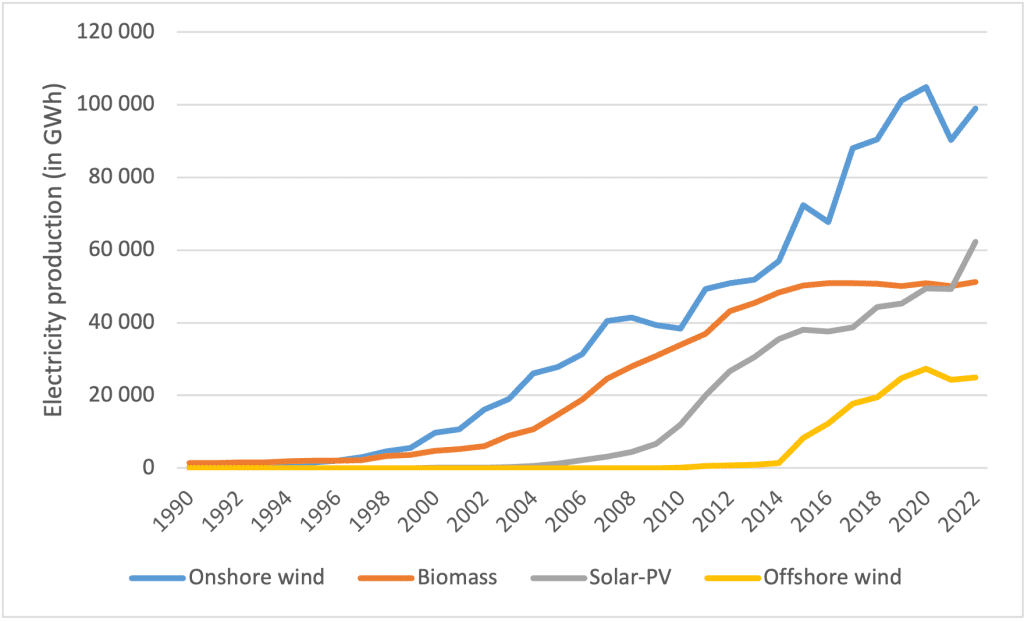
Although one can always quibble about the precise demarcation of transition phases, the unfolding German electricity transition has so far progressed through three periods. In the first period (1986-1998), niche-innovations were nurtured in the context of stable regimes. Wind turbines and solar-PV were supported by R&D programs introduced after the oil crises in the 1970s, but deployment remained limited in the 1980s because of poor performance and high costs (Jacobsson and Lauber, 2006). The 1986 Chernobyl accident was a landscape shock that stimulated some deployment of wind turbines by new entrants such as environmentally motivated citizens, farmers, and anti-nuclear activists who wanted to demonstrate the feasibility of alternatives. The accident also created negative public attitudes towards nuclear power, which continued to be supported, however, by successive Conservative-Liberal governments.
Several proposals for RET market support were defeated in Parliament, but the 1990 proposal succeed ‘by accident’ as the government was preoccupied with German re-unification (Jacobsson and Lauber, 2006). It was not expected that the resulting Feed-In-Law would have major effects and, in 1994, the Minister of Environmental Affairs (Angela Merkel) thought it unlikely that Germany would ever generate more than 4% renewable electricity (Lauber and Jacobsson, 2016). But the Feed-In-Law, which obliged utilities to purchase renewable electricity at 90% of the retail price, made onshore wind deployment economically feasible and stimulated significant deployment in the 1990s (Figure 5). The success of German turbine manufacturers also attracted industrial policy support in the peripheral regions of Northern Germany, which expanded the RET advocacy coalition (Geels et al., 2016).
To hinder RETs, incumbent utilities lobbied the government, which in 1997 proposed to reduce feed-in tariffs. But public protests by the RET advocacy coalition (including environmental groups, solar and wind associations, metal and machine workers, farmer groups, and church groups) led to the rejection of the proposal by the German Parliament (Jacobsson and Lauber, 2006).
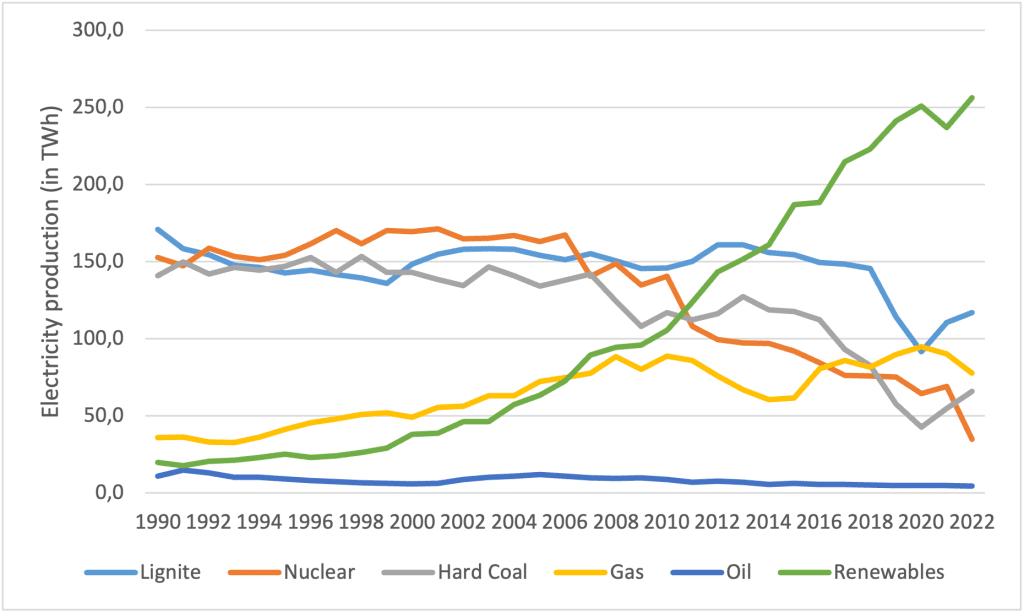
In the second period (1998-2009), the election of a ‘Red-Green’ coalition government between the Social Democratic Party and the Green Party (1998-2005) was another landscape shock, which disrupted the cosy regime-level relations between utilities and policymakers (Geels et al., 2016). The new government decided to phase-out nuclear energy and support RETs with the Renewable Energy Act, which guaranteed fixed, premium payments for renewable electricity over a 20-year period, with tariffs varying with the maturity of the technology.
Renewable electricity subsequently diffused rapidly from 6.6% in 2000 to 15.9% in 2009 (Figure 4), because of reinforcing developments in multiple environments. In the policy environment, generous and stable feed-in tariffs created attractive market opportunities. In the business environment, new entrants (like households, farmers, municipal utilities, project developers, and other industries) dominated RET-deployment, while the incumbent utilities produced only 6.5% of renewable electricity in 2010. The very rapid diffusion of solar-PV after 2006 (Figure 5) was unforeseen and driven by feed-in-tariffs that exceeded generation cost as the price of solar-PV panels decreased rapidly. This stimulated strong interest from households, who deployed small-scale rooftop PV systems, and from farmers, who deployed large-scale roof- and field-mounted systems (Dewald and Truffer, 2011). Solar-PV became an industrial success story, as total sales of the German PV industry grew from €201 million in 2000 to €7 billion in 2008. Export sales grew from €273 million in 2004 to approximately €5 billion in 2010 (BSW-Solar, 2010). In the public domain, broad advocacy coalitions and positive discourses about renewable energy, ecological modernisation and green growth supported and legitimated RET-diffusion and policy support (Geels et al., 2016).
Instead of addressing renewable energy, incumbent regime actors focused on other issues. Liberalization of the electricity sector in 1998 triggered a wave of mergers and acquisitions, which resulted in the Big-4 utilities (RWE, E.ON, Vattenfall, EnBW) that captured 90% of the wholesale market by 2004. By the mid-2000s, the Big-4 were investing in new coal- and gas-fired power plants to meet expected demand growth (Kungl and Geels, 2018). They also focused on European and global expansions, which boosted growth and stock prices. After years of lobbying, the utilities also scored a political victory when the newly elected (2009) Conservative-Liberal government decided to overturn the earlier nuclear phase-out decision.
In the third period (2009-2022), RETs further diffused because of feed-in-tariffs, positive discourses, and declining RET-prices. Between 2010 and 2020, the global average levelised cost of electricity decreased by 85% for utility-scale solar-PV, 56% for onshore wind and 48% for offshore wind (Figure 6).
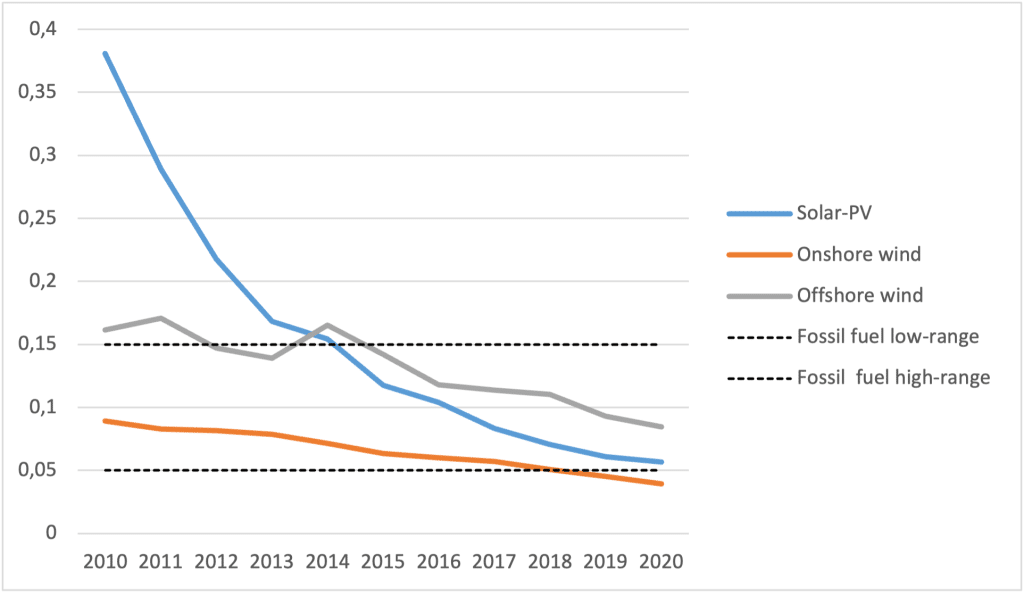
RET-diffusion was also facilitated by a landscape shock (the 2011 Fukushima accident), which destabilized the regime because the government performed a U-turn and re-introduced a nuclear phase-out policy, with a target date of 2022. The government also adopted an official energy transition policy (Energiewende) that included ambitious future targets for renewable electricity (35% by 2020, 40-45% by 2025, 55-60% by 2035, and 80% by 2050).
The existing regime destabilised and experienced various problems in this period (Geels et al., 2016): a) the expansion of renewables reduced the market share of existing fossil plants and decreased wholesale electricity prices because of the ‘merit order effect’ (meaning that solar-PV and wind, with low marginal costs, were dispatched first in power generation), b) the aftermath of the 2007/8 financial crisis (another landscape shock) depressed economic activity and reduced electricity demand, which eroded the economic viability of the newly build fossil plants, c) the nuclear phase-out decision implied write-off costs. These developments reduced net incomes of the Big-4 utilities after 2011 and created doubts about the viability of traditional business models. Consequently, incumbent utilities began strategic reorientation activities (Kung and Geels, 2018). In 2014, E.ON split into two companies: one focused on renewables, distribution grids and service activities; the other holding conventional assets in large-scale electricity production and trading activities. In 2015, Vattenfall offered its German lignite activities for sale. And in 2015 RWE announced plans to separate its renewables, grid and retail business into a new sub-company.
Diffusing RETs also experienced several unforeseen problems (Geels et al., 2016): a) many German PV-manufacturers went bankrupt because of increasing imports of cheaper Chinese solar panels; this eroded the salience of the green growth discourse; b) renewables deployment (especially solar-PV) increased EEG-surcharges from 1.3 eurocent/kWh in 2009 to 6.24 eurocent/kWh in 2014, making German retail electricity prices the highest in Europe, c) the increasing surcharges provided ammunition for political opposition from utilities and the Economics Ministry; and d) intermittent renewables threatened grid stability and increased price volatility, leading to negative prices on sunny, windy days when supply exceeded demand.
These RET-related problems and the economic problems of utilities (which were seen as ‘too big to fail’) led to government efforts to slow RET-expansion and increase support for the utilities: a) feed-in-tariffs were reduced in several rounds (Hoppmann et al., 2014), b) from 2017 onwards, feed-in-tariffs were replaced by a bidding system for target capacity (which required capabilities and resources that suited big players), c) offshore wind deployment was stimulated, which provided attractive diversification opportunities for incumbents because of size and cost structures.
Recent landscape shocks such as the COVID-19 pandemic, Putin’s war in Ukraine, and the energy crisis (particularly for gas) have not disrupted the diffusion of RETs, although the latter two shocks did lead to increased use of hard coal and lignite (Figure 4, 5). While increased coal use is expected to be temporary, German and European policymakers have further increased RET-targets and support schemes, which are expected to accelerate the low-carbon electricity transition in the coming years.
This brief case study shows that the German electricity transition resonates very well with the phases, levels, and conceptual dimensions of the MLP, which together with many other case studies have enhanced the framework’s empirical robustness and analytical appeal.
4. Further thematic developments and current research topics
In the past decade, the MLP has been further developed in response to criticisms and through interactions with hundreds of MLP-based case studies. In response to early criticisms about over-emphasis on bottom-up substitution processes, one important further development has been the articulation of different transition pathways (Geels and Schot, 2007; Geels et al., 2016; Rosenbloom, 2017), based on types and sequences of multi-level interactions. Transition pathways include not only the basic substitution pattern of Figure 3 (in which niche-innovations replace (parts of) the existing system) but also transformation (in which incumbent actors gradually adjust the existing system and regime to accommodate landscape pressures), reconfiguration (in which symbiotic niche-innovations are incorporated into the existing system, followed by knock-on effects that gradually alter the system architecture) and de-alignment and re-alignment (in which strong landscape pressures destabilise the system, which creates space for multiple emerging niche-innovation, followed by re-alignment of a new system around one of them). The substitution pathway is increasingly considered the exception rather than the rule, especially if one is interested in whole system change, which is more likely to follow a reconfiguration pattern, as will be discussed further below (McMeekin et al., 2019; Bui, 2021; Geels and Turnheim, 2022; Andersen et al., 2023a).
In response to early criticisms about lack of agency, another major development has been the elaboration of various actor roles and agentic processes in socio-technical transitions (see also Chapters 17 and 20 in this book). Mobilising concepts from political science, cultural discourse theory, business studies, and other social science disciplines, transition scholars have elaborated the MLP by further conceptualising varying roles, sub-processes, and temporal phases with regard to politics and power (Kern and Rogge, 2018; Roberts and Geels, 2018), discursive framing struggles (Rosenbloom et al., 2016), grassroots innovation and community initiatives (Seyfang et al., 2014), intermediary actors (Kivimaa et al., 2019), users (Schot et al., 2016), and incumbent firm reorientation (Bergek et al., 2013). This research strand continues to be important and vibrant because actors engage in many different types of activities, which often vary between systems and countries.
Transition scholars have also identified new topics, which invited new conceptualisations. One topic is niche-regime interaction (see also Chapter 9 in this book), which has led to more differentiated understandings of multi-level interactions. Smith (2007) identified ‘translation’ as an important process through which elements of niche-innovations are selectively appropriated into established regimes. Smith and Raven (2012) further emphasised the importance of niche ‘empowerment’, which are externally-oriented activities through which niche advocates aim to change rules and selection criteria in socio-technical regimes. This concept led them to distinguish two kinds of diffusion and niche-regime interaction patterns: ‘fit-and-conform’ (in which niche-innovations diffuse because they fit in existing selection environments) and ‘stretch-and-transform’ (in which niche-innovations diffuse because advocates succeed in transforming existing regimes). Diaz et al. (2013) further suggested that niche actors can attempt to enrol regime actors with more resources to help further develop niche-innovations, while Ingram (2018) highlighted the roles of knowledge flows from niche-innovations into regimes (via certification, standardization, networking, learning, and frame linkage). This research topic remains important, because its multi-dimensional approach offers important correctives to the techno-economic approaches that dominate mainstream sustainability and policy debates.
A second new topic that has invited conceptual elaborations is regime destabilisation, decline and phase-out, which can be seen as the flipside of transitions (Turnheim and Geels, 2013; Kungl and Geels, 2018). Work on this topic not only aims to correct the innovation bias in transitions research, but also emphasizes that addressing time-constrained sustainability problems may require deliberate phase-out policies to increase the speed of change (Rogge and Johnstone, 2017). This research strand is becoming more important as the diffusion of niche-innovations like solar-PV, wind turbines, and electric vehicles is increasingly causing the decline of existing technologies like coal and internal combustion vehicles, while deliberate phase-out policies are also being implemented or considered (e.g., for incandescent lightbulbs, diesel and petrol cars, nuclear power, gas boilers).
Thirdly, diffusion and acceleration are increasingly important topics (see also Chapter 8 in this book), leading to increasing research interest in the drivers and challenges in the shift from phase 2 to phase 3 in the MLP (Geels and Johnson, 2018; Markard et al., 2020). Although diffusion has been studied for decades with relatively simple adoption models, analysis of this topic for sustainability transitions throws up new research puzzles because of the important role of socio-political drivers in shaping markets and supporting innovations. Analyses of solar-PV, wind turbines, and electric vehicles (Markard and Hoffman, 2016; Kern et al., 2019; Geels and Ayoub, 2023) shows that rapid diffusion usually stems from the interactions between multiple processes, including: a) technological performance improvements, resulting from R&D activities, learning-by-doing, and complementary innovations, b) cost reductions resulting from scale economies, improved manufacturing, and lower financing costs, c) increasing interests from consumers as preferences change or new technologies become better or cheaper, d) societal debates, which shape consumer preference and policy agendas, e) increasing confidence and commitment by companies, leading to increased investments and marketing, f) stronger policy support for innovations through R&D subsidies, purchase subsidies, regulations, direct infrastructure investment, often in response to public debates and industrial lobbies.
This research topic is increasingly important, because meeting agreed policy targets (for climate change, biodiversity, or sustainable development goals) will require acceleration of many niche-innovations. Additionally, real-world acceleration of some innovations is giving rise to innovation races where companies and countries are increasingly competing for future markets and industries. The Inflation Reduction Act, for example, which the United States introduced in 2022, is widely seen as a game changer because of the commitment and large sums ($369 billion over 10 years) that are being allocated to supporting the development and deployment of carbon capture and storage, green hydrogen, electrolysers, fuel cells, solar-PV, batteries, wind turbines, electric vehicles, and heat pumps. In response, the European Commission rushed out its Green Deal Industrial Plan for the Net-Zero Age in 2023, which aims to support similar innovations in various ways, giving rise to industrial policy competition that is likely to accelerate net-zero transitions.
A fourth new topic that is attracting more attention is multi-system interaction (see also Chapter 10 in this book). Early research (Geels, 2007; Raven and Verbong, 2007) explored the phenomenon and proposed basic MLP-distinctions (such as regime-regime, niche-niche, and regime-niche interactions between different systems) and types of interaction such as competition, symbiosis, integration, and spillover. Increasing commitments in recent years to net-zero targets have stimulated a new wave of research on the topic (Rosenbloom, 2020; Andersen et al., 2023b), because reaching these targets will require interacting transitions across mobility, heating, buildings, electricity, agri-food, and industrial systems as they will require inputs from or have effects on other systems. Current research aims to better understand the causal drivers and barriers in the material flows between systems, actor diversification from one system to another, the role of pervasive technologies, and institutional (mis)alignment between systems (Andersen and Geels, 2023).
A fifth new topic is whole system reconfiguration (McMeekin et al., 2019; Bui, 2021; Geels and Turnheim, 2022; Andersen et al., 2023a). Research on this topic takes more serious the idea that systems consist of multiple sub-systems and components that can change sequentially. The electricity system, for example, consists not just of electricity generation, but also of a distribution sub-system (e.g., transmission and distribution grids) and a consumption sub-system, which are each characterised by different actors, institutions, and technologies (McMeekin et al., 2019). Whole system transitions thus involve multiple regimes and multiple niche-innovations, which interact in many ways (Bui, 2021). Component changes in one sub-system (e.g., solar-PV, wind turbines, bio-power replacing coal-fired power generation) can then trigger knock-on effects in other sub-systems (e.g., smart grids, battery storage, and demand-side response in distribution and consumption sub-systems to address intermittency issues). Conceptualisations of whole system reconfiguration thus change the transition imagery from bottom-up substitution patterns to more gradual reconfiguration processes involving successive changes in technical components, institutional adjustments, and changes in actor’s views and strategies (Geels and Turnheim, 2022).
A new sixth topic that is gaining more attention is incumbent reorientation. This is partly a corrective of early transitions research, which arguably presented transitions too much in ‘David-versus-Goliath’ terms, over-emphasizing the importance of outsiders, start-ups, entrepreneurs, and grassroots actors in developing niche-innovations and challenging regimes. While early transitions research presented incumbents mostly in terms of lock-in, inertia, and resistance, subsequent empirical research showed that incumbent firms can reorient their perceptions, strategies and investments towards niche-innovations like electric vehicles, trams, wind parks, bio-electricity, light emitting diodes, smart grids, and battery storage (Bergek et al., 2013; Berggren et al., 2015; Apajalahti et al., 2018; Turnheim and Geels, 2019; Kattirtzi et al., 2021; Geels and Ayoub, 2023). This reorientation typically involves pressures from policymakers, markets, and civil society, and progresses through several phases (including hedging and diversification). Although incumbent reorientation is not an easy process, it can accelerate sustainability transitions when incumbents mobilise their large financial, technical, and organizational resources.
A seventh new topic is increasing attention for potential trade-offs between the speed and depth of change (Geels and Turnheim, 2022; Newell et al., 2022). The empirical evidence indicates that accelerating niche-innovations are mostly technologies (e.g., electric vehicles, solar-PV, wind turbines) that do not require the overhaul of existing systems. Niche-innovations that imply ‘deeper’ change and social innovation (e.g., car sharing, mobility-as-a-service, agroecology, passive house, whole-house retrofit) have remained small and appear to limitedly diffuse or scale-up. The reason is that modular technical change is easier, less disruptive, and better fits the interests of incumbent firms, mainstream consumers, and policymakers. While many sustainability transitions scholars have a normative preference for the deepest, most radical kinds of innovations, real-world developments in recent years suggest that the transitions community should investigate more deeply the feasibility of change at the scale and speed required. Instead of repeating calls for ‘deeper’ change, it may be more fruitful to investigate how and under which conditions rapid changes in system modules can have knock-on effects that trigger deeper whole system reconfiguration.
In sum, MLP-based transition research has been generative in identifying new topics and asking new kinds of questions, which stems from a desire and willingness to follow and reflect on real-world developments that throw up new puzzles as sustainability transitions are unfolding and accelerating. Since different questions require different conceptual tools, the MLP has continued to evolve conceptually and empirically, which has enabled it to become a generative research program that continues to appeal to a broadening group of academics (and policymakers, which this brief chapter was unable to discuss)
Andersen, A. D., Markard, J., Bauknecht, D., Korpås, M., 2023a, Architectural change in accelerating transitions: Actor preferences, system architectures, and flexibility technologies in the German energy transition, Energy Research & Social Science, 97, 102945.
Andersen, A.D., Geels, F.W., Steen, M., Bugge, M., 2023b, Building multi-system nexuses in low-carbon transitions: Conflicts and asymmetric adjustments in Norwegian ferry electrification, Proceedings of the National Academy of Sciences, 120(47), e2207746120.
Andersen, A.D., and Geels, F.W., 2023, Multi-system dynamics and the speed of net-zero transitions: Identifying causal processes related to technologies, actors, and institutions, Energy Research & Social Science, 102, 103178.
Apajalahti, E-L., Temmes, A., Lempiälä, T., 2018, Incumbent organisations shaping emerging technological fields: Cases of solar photovoltaic and electric vehicle charging, Technology Analysis & Strategic Management, 30(1), 44-57.
Bergek, A., Berggren, C., Magnusson, T., Hobday, M., 2013, Technological discontinuities and the challenge for incumbent firms: Destruction, disruption or creative accumulation?, Research Policy, 42(6-7), 1210-1224.
Berggren, C., Magnusson, T., Sushandoyo, D., 2015, Transition pathways revisited: Established firms as multi-level actors in the heavy vehicle industry, Research Policy, 44(5), 1017-1028.
Bertels, K., 1973. Geschiedenis Tussen Struktuur en Evenement: Een Methodologies en Wijsgerig Onderzoek. (‘History Between Structure and Event: A Methodological and Philosophical Investigation’) Wetenschappelijke Uitgeverij BV, Amsterdam.
Braudel, F., 1970, History and the social sciences: The long term’, Social Science Information, 9(1), 145-174.
BSW-Solar, 2010, Statistical data on the German photovoltaic industry, German Solar Industry Association
(http://en.solarwirtschaft.de/fileadmin/content_files/factsheet_pv_engl.pdf, accessed 31th May 2011).
Bui, S., 2021, Enacting transitions: The combined effect of multiple niches in whole system reconfiguration, Sustainability, 13, 6135.
Dewald, U., Truffer, B., 2011, Market formation in technological innovation systems: Diffusion of photovoltaic applications in Germany, Industry and Innovation, 18(3), 285-300.
Diaz, M., Darnhofer, I., Darrot, C., Beuret, J-E., 2013, Green tides in Brittany: What can we learn about niche-regime interactions?, Environmental Innovation and Societal Transitions, 8, 62-75.
EEA, 2019, Sustainability Transitions: Policy and Practice, EEA Report 09/2019, European Environment Agency, Copenhagen.
Fuenfschilling, L., Truffer, B., 2014, The structuration of socio-technical regimes: Conceptual foundations from institutional theory, Research Policy, 43(4), 772-791.
Geels, F.W., 2002, Technological transitions as evolutionary reconfiguration processes: A multi-level perspective and a case-study, Research Policy, 31(8-9), 1257-1274.
Geels, F.W., 2004, From sectoral systems of innovation to socio-technical systems: Insights about dynamics and change from sociology and institutional theory, Research Policy, 33(6-7), 897-920.
Geels, F.W., Raven, R.P.J.M., 2006, Non-linearity and expectations in niche-development trajectories: Ups and downs in Dutch biogas development (1973-2003), Technology Analysis & Strategic Management, 18(3/4), 375-392.
Geels, F.W., 2007, Analysing the breakthrough of rock ‘n’ roll (1930-1970): Multi-regime interaction and reconfiguration in the multi-level perspective, Technological Forecasting and Social Change, 74(8), 1411-1431.
Geels, F.W., Schot, J.W., 2007, Typology of sociotechnical transition pathways, Research Policy, 36(3), 399-417.
Geels, F.W., McMeekin, A., Mylan, J., Southerton, D., 2015, A critical appraisal of Sustainable Consumption and Production research: The reformist, revolutionary and reconfiguration positions, Global Environmental Change, 34, 1-12.
Geels, F.W., 2014, Regime resistance against low-carbon energy transitions: Introducing politics and power in the multi-level perspective, Theory, Culture & Society, 31(5), 21-40.
Geels, F.W., Kern, F., Fuchs, G., Hinderer, N., Kungl, G., Mylan, J., Neukirch, M., Wassermann, S., 2016, The enactment of socio-technical transition pathways: A reformulated typology and a comparative multi-level analysis of the German and UK low-carbon electricity transitions (1990-2014), Research Policy, 45(4), 896-913.
Geels, F.W., Johnson, V., 2018, Towards a modular and temporal understanding of system diffusion: Adoption models and socio-technical theories applied to Austrian biomass district-heating (1979-2013), Energy Research and Social Science, 38, 138-153.
Geels, F.W., 2019, Socio-technical transitions to sustainability: A review of criticisms and elaborations of the Multi-Level Perspective, Current Opinion in Environmental Sustainability, 39, 187-201.
Geels, F.W., 2020a, Transformative innovation and socio-technical transitions to address grand challenges, in: Science, Research and Innovation Performance of the EU 2020: A Fair, Green and Digital Europe, DG Research and Innovation, European Commission, Brussels, p. 572-607.
Geels, F.W., 2020b, Micro-foundations of the Multi-Level Perspective on socio-technical transitions: Developing a multi-dimensional model of agency through crossovers between social constructivism, evolutionary economics and neo-institutional theory, Technological Forecasting and Social Change, 152, 119894.
Geels, F.W., 2022, Causality and explanation in socio-technical transitions research: Mobilising epistemological insights from the wider social sciences, Research Policy, 51(6), 104537.
Geels, F.W., Turnheim, B., 2022, The Great Reconfiguration: A Socio-Technical Analysis of Low-Carbon Transitions in UK Electricity, Heat, and Mobility Systems, Cambridge University Press.
Geels, F.W., Ayoub, M., 2023, A socio-technical transition perspective on positive tipping points in climate change mitigation: Analysing seven interacting feedback loops in offshore wind and electric vehicles acceleration, Technological Forecasting and Social Change, 193, 122639.
Genus, A., Coles, A-M., 2008, Rethinking the multi-level perspective of technological transitions, Research Policy, 37(9), 1436-1445.
Hansmeier, H., Schiller, K., Rogge, K.S., 2021, Towards methodological diversity in sustainability transitions research? Comparing recent developments (2016-2019) with the past (before 2016), Environmental Innovation and Societal Transitions, 38, 169-174.
Holtz, G., Brugnach, M., Pahl-Wostl, C., 2008, Specifying “regime”: A framework for defining and describing regimes in transition research, Technological Forecasting and Social Change, 75(5), 623-643.
Hoppmann, J., Huenteler, J., Girod, B., 2014. Compulsive policy-making: The evolution of the German feed-in tariff system for photovoltaic power, Research Policy, 43(8), 1422–1441.
Ingram, J., 2018, Agricultural transition: Niche and regime knowledge systems’ boundary dynamics, Environmental Innovation and Societal Transition, 26, 117-135.
IRENA, 2021, Renewable Power Generation Costs in 2020, International Renewable Energy Agency, Abu Dhabi.
Jacobsson, S., Lauber, V., 2006, ‘The politics and policy of energy system transformation: Explaining the German diffusion of renewable energy technology’, Energy Policy, 34(3), 256-276.
Kattirtzi, M., Ketsopoulou, I., Watson, J., 2021, Incumbents in transition? The role of the ‘Big Six’ energy companies in the UK, Energy Policy, 148, Part A, 111927.
Kern, F., Rogge, K., 2018, Harnessing theories of the policy process for analysing the politics of sustainability transitions: A critical survey, Environmental Innovation and Societal Transition, 27, 102-117.
Kern, F., Rogge, K., Howlett, M., 2019, Policy mixes for sustainability transitions: New approaches and insights through bridging innovation and policy studies, Research Policy, 48(10), 103832.
Kivimaa, P., Hyysalo, S., Boon, W., Klerkx, L., Martiskainen, M., Schot, J., 2019, Passing the baton: How intermediaries advance sustainability transitions in different phases. Environmental Innovation and Societal Transitions, 31, 110-125
Klitkou, A., Bolwig, S., Hansen, T., Wessberg, N., 2015, The role of lock-in mechanisms in transition processes: The case of energy for road transport, Environmental Innovation and Societal Transitions, 16, 22-37.
Kungl, G., Geels, F.W., 2018, Sequence and alignment of external pressures in industry destabilization: Understanding the downfall of incumbent utilities in the German energy transition (1998-2015), Environmental Innovation and Societal Transition, 26, 78-100.
Lauber, V., Jacobsson, S., 2016, The politics and economics of constructing, contesting and restricting socio-political space for renewables – The German Renewable Energy Act, Environmental Innovations and Societal Transitions, 18, 147–163.
Markard, J., Hoffmann, V.H., 2016, Analysis of complementarities: Framework and examples from the energy transition, Technological Forecasting and Social Change, 111, 63-75.
Markard, J., Geels, F.W., Raven, R. 2020, Challenges in the acceleration of sustainability transitions, Environmental Research Letters, 15(8), 081001.
McMeekin, A., Geels, F.W., Hodson, M., 2019, Mapping the winds of whole system reconfiguration: Analysing low-carbon transformations across production, distribution and consumption in the UK electricity system, Research Policy, 48(5), 1216-1231
Meadowcroft, J., 2009, What about the politics? Sustainable development, transition management, and long term energy transitions, Policy Sciences, 42(4), 323-340.
Nelson, R.R., 2008, Bounded rationality, cognitive maps, and trial and error learning, Journal of Economic Behaviour & Organization, 67(1), 78-89.
Newell, P., Geels, F.W., Sovacool, B.K., 2022, Navigating tensions between rapid and just low-carbon transitions, Environmental Research Letters, 17(4), 041006.
Oudshoorn, N., Pinch, T. (Eds.): How Users Matter: The Co-Construction of Users and Technology. MIT Press; 2003.
Penna, C.C.R., Geels, F.W., 2015, Climate change and the slow reorientation of the American car industry (1979-2011): An application and extension of the Dialectic Issue LifeCycle (DILC) model, Research Policy, 44(5), 1029-1048.
Raven R.P.J.M., Verbong G.P.J., 2007, Multi-regime interactions in the Dutch energy sector. The case of combined heat and power in the Netherlands 1970-2000, Technology Analysis and Strategic Management, 19(4), 491-507.
Rip, A., 1992, A quasi-evolutionary model of technological development and a cognitive approach to technology policy, Rivista di Studi Epistemologici e Sociali Sulla Scienza e la Tecnologia, Vol. 2, 69-103.
Rip, A., Kemp, R., (1996): Towards a Theory of Socio-Technical Change, mimeo Twente University, Report prepared for Batelle Pacific Northwest Laboratories, Washington, D.C.
Roberts, C., Geels, F.W., 2018, Public storylines in the British transition from rail to road transport (1896-2000): Discursive struggles in the Multi-Level Perspective, Science as Culture, 27(4), 513-542.
Roberts, C., Geels, F.W., 2019, Conditions for politically accelerated transitions: Historical institutionalism, the multi-level perspective, and two historical case studies in transport and agriculture, Technological Forecasting and Social Change, 140, 221-240.
Rogge, K.S., Johnstone, P., 2017, Exploring the role of phase-out policies for low-carbon energy transitions: The case of the German Energiewende, Energy Research & Social Science, 33, 128-137.
Rosenbloom, D., Berton, H., Meadowcroft, J., 2016, Framing the sun: A discursive approach to understanding multi-dimensional interactions within socio-technical transitions through the case of solar electricity in Ontario, Canada, Research Policy, 45(6), 1275–1290.
Rosenbloom, D., 2017, Pathways: An emerging concept for the theory and governance of low-carbon transitions, Global Environmental Change, 43, 37-50.
Rosenbloom, D., 2020, Engaging with multi-system interactions in sustainability transitions: A comment on the transitions research agenda, Environmental Innovation and Societal Transitions, 34, 336-340.
Schot, J.W., 1992, The policy relevance of the quasi-evolutionary model: The case of stimulating clean technologies, In: Coombs, R., Saviotti, P., Walsh, V. (Eds.), Technological Change and Company Strategies: Economic and Sociological Perspectives, London: Academic Press, pp. 185-200.
Schot, J.W., Geels, F.W., 2008, Strategic niche management and sustainable innovation journeys: Theory, findings, research agenda and policy, Technology Analysis & Strategic Management, 20(5), 537-554.
Schot, J., Kanger, L., Verbong, G.P.J., 2016, The roles of users in shaping transitions to new energy systems, Nature Energy, 1(5), 16054.
Seyfang, G., Hielscher, S., Hargreaves, T., Martiskainen, M., Smith, A., 2014, A grassroots sustainable energy niche? Reflections on community energy in the UK, Environmental Innovation and Societal Transitions, 13, 21-44.
Smith, A., 2007, Translating sustainabilities between green niches and socio-technical regimes, Technology Analysis & Strategic Management, 19(4), 427-450.
Smith, A., Raven, R., 2012, What is protective space? Reconsidering niches in transitions to sustainability, Research Policy, 41(6), 1025-1036.
Turnheim, B., Geels, F.W., 2013, The destabilisation of existing regimes: Confronting a multi-dimensional framework with a case study of the British coal industry (1913-1967), Research Policy, 42(10), 1749-1767.
Turnheim, B., Geels, F.W., 2019, Incumbent actors, guided search paths, and landmark projects in infra-system transitions: Rethinking Strategic Niche Management with a case study of French tramway diffusion (1971-2016), Research Policy, 48(6), 1412-1428.
Walker, W., 2000, Entrapment in large technology systems: Institutional commitments and power relations, Research Policy, 29(7-8), 833-846.